Where to search for signs of life

A recent study accepted for publication in the Astrophysical Journal proposes a new strategy for searching for biosignatures, chemical evidence of the presence of life on exoplanets. CEHW graduate student Noah Tuchow and professor Jason Wright have developed a theoretical framework to determine which stars have a higher chance of hosting biosignatures on their planets. This framework is based on the long-term habitability of exoplanets, and how long a planet remains in its star’s habitable zone as the star and its habitable zone evolve over time.
Habitable zones around stars are defined as the range of distances where an Earth-like planet could support liquid water on its surface, provided it has a thick enough atmosphere. The luminosity of a star plays a key role in defining the habitable zone. As stars evolve over their lifetimes, they typically become more luminous and their habitable zones move outwards. When we discover new planets, we would like to know how long they have been in the habitable zone and whether life has had time to emerge. We would also like to know whether “cold start” planets, originating outside the habitable zone but entering it as the star becomes brighter, could be habitable.
Considering long-term habitability is vitally important if one would like to find planets where life has had time to originate and develop. In the case of the roughly 4.5 billion year history of Earth, life is thought to have emerged early in the planet’s lifetime. However, oxygen didn’t appear as a biosignature in the Earth’s atmosphere until around 2 billion years after that. Even then, oxygen levels in the atmosphere may have been much lower, and potentially undetectable to a remote observer, up until the end of the Proterozoic eon, roughly 4 billion years after the formation of the Earth. If life on other planets evolves on similar timescales to that of Earth, it makes sense to search for observable signs of life on older planets that have remained habitable for a long duration. Most nearby Sun-like stars are between 1-10 billion years old, but if life on their planets evolves on Earth-like timescales, then one would only want to search for life around the fraction of stars with ages exceeding 4 billion years.
This study developed a means to measure the long-term habitability of planets around other stars and their potential to host biosignatures. It defines a quantity called the biosignature yield, B, which quantifies how likely it is that a given star hosts a planet with biosignatures. B depends on two functions: how Earth-like planets are typically distributed in distance, and how likely it is that biosignatures emerge. We don’t know either of these very well, but if they are the same for two stars, we can determine the relative likelihood between the two stars of hosting biosignatures. For instance, as long as biosignatures are more likely to emerge the older the planet is, then we would expect to find more biosignatures around older planets.
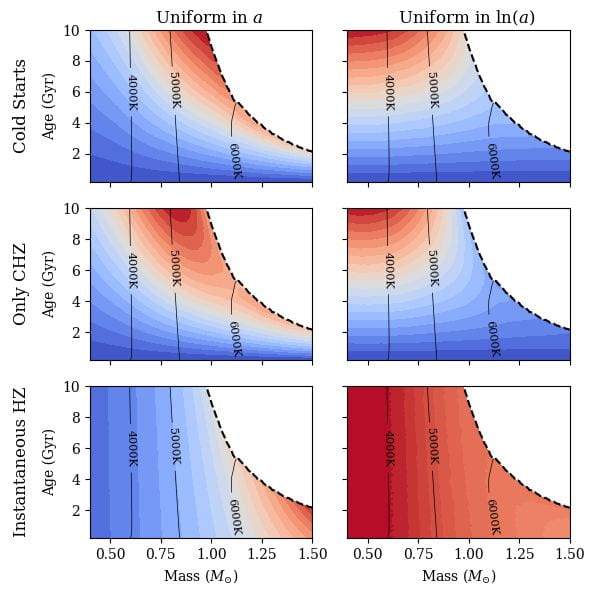
Figure 1. Biosignature yields for different stellar masses and ages. Rows are assumptions about the emergence of life, and columns are assumptions about the distribution of planets. Color scale goes from highest in red to zero in blue.
With different assumptions about the form of the functions that determine B, this study shows that different populations of stars are favored in terms biosignature yields. Tuchow and Wright ran stellar models with different masses and ages and observed how biosignature yields changed in response. They found that if the probability of biosignatures emerging depends on the time spent in the habitable zone, then older low mass stars have a high chance of hosting detectable biosignatures. If only planets that stay in the habitable zone for their entire lifetime can host life, then less massive, K stars are preferred, while if cold start planets can be considered habitable then slightly more massive stars are favored. Assuming that any planet in the current day, instantaneous habitable zone has the same chance of hosting biosignatures can lead astronomers to misleading conclusions, and may favor more massive, luminous stars, despite their major obstacles to habitability.
The authors of this study intend this framework to be used in the context of missions to directly image planets around other stars. Because stars are extremely bright compared to their planets, it is a major challenge to block out a star’s light and observe the planets directly. Nevertheless, future missions such as HabEx and LUVOIR aim to extend our current level of technology and directly image Earth-sized planets in the habitable zones of their stars. These missions aim to study the atmospheres of Earth-like exoplanets, and by using their spectra to infer their compositions, we will be able to detect biosignatures if they are present. However, since it takes time for telescopes to obtain an accurate spectrum of a planet, these missions will need to choose which stars to observe and prioritize.
The framework developed in this study could help motivate which stars will be prioritized by future missions. To demonstrate that stellar properties are known to a high enough precision for biosignature yields to be useful, this study looked at two well studied example stars: 55 Cancri and Theta Cygni. For both stars, the biosignature yields can be determined to a precision sufficient to rank which star is a better candidate in a search for life. For treatments of biosignature yield using different assumptions about the occurrence rates of planets and the emergence of life, they showed that 55 Cancri, a small older star, was a much better candidate for hosting biosignatures than Theta Cygni, a more massive, younger star.

Figure 2. Comparison of biosignature yields between 55 Cancri and Theta Cygni. CHZ refers to the continuously habitable zone.
Tuchow and Wright plan to incorporate this framework in future studies about the effects of stellar evolution on planetary habitability and target selection for exoplanet direct imaging missions. A preprint of the paper can be found at https://arxiv.org/abs/2010.13762.
Source: Noah Tuchow